Imaging of Thyrotoxicosis
Abstract
The diagnostic algorithm for patients with Graves’ disease frequently involves the use of thyroid autoantibody testing and radioisotope scanning. However, ultrasound has myriad uses in the evaluation of patients with hyperthyroidism. The purpose of this paper is to review the medical literature outlining the important role that ultrasonography (US) can play in the diagnosis and management of patients with hyperthyroidism. Further, we aim to make a case for the use of ultrasonography in the initial evaluation of all patients with thyrotoxicosis.A highly sensitive and specific test for diagnosing various causes of hyperthyroidism, US has an accuracy rate closely approximating that of nuclear scintigraphy. We will delineate the advantages of ultrasonography compared to nuclear medicine imaging. Serial ultrasonographic exams using traditional US techniques and color-flow Doppler sonography (CFDS) after initiation of medical therapy can help predict relapse or remission of Graves’ disease, aiding the clinician in determining the best form of therapy. An additional tool available on most modern US machines is color-flow Doppler sonography, a device that measures the vascularity in the area being imaged. Information gathered from this exam may help delineate the cause of the hyperthyroidism and may be used to predict remission of Graves’ disease after initiation of medical therapy. Finally, this paper will detail the importance of ultrasonography in the workup of hyperthyroidism during pregnancy. We will also outline its utility for predicting fetal thyroid dysfunction in maternal Graves’ disease without the need for cordocentesis and its attendant complications.
Introduction
The use of ultrasonography (US) for the evaluation of thyroid disorders was first described in the mid-1960s[1] but it has gained more widespread acceptance as an important diagnostic tool in the last 15-20 years[2]. The superficial location of the gland in the anterior neck and the ability to visualize multiple planes real-time make US an ideal imaging modality for thyroid disorders. With recent advances in technology, most US probes have excellent spatial resolution; nodules as small as 2-3mm may be readily distinguished with modern machines. Further, the addition of color Doppler sonography to new devices improves the diagnostic capacity for clinicians at the bedside through its ability to quantify vascularity in the gland. Recognition of its superior diagnostic accuracy has led to a trend of increasing reliance on US at the bedside among Endocrinologists. Ultrasound training increasingly has become a part of the educational curriculum for Endocrine fellows. While it is most commonly used for the detection of thyroid nodules and guidance for fine-needle aspirations (FNAs), ultrasound is also an important tool in the initial workup of patients with hyperthyroidism.There are numerous causes of hyperthyroidism, the two most common are Graves’ disease (GD) and toxic adenoma(s). Because only 50% of thyroid nodules measuring between 1-2cm are palpable[3], distinguishing between Graves’ disease and toxic nodules sometimes can be challenging. Other, less frequent, causes of hyperthyroidism include the various types of destructive thyroiditis. This paper will outline the characteristic ultrasonographic features of each of these conditions and how US can help predict outcomes after treatment of Graves’ disease. We will also explain the applications of US during pregnancy for the noninvasive monitoring of maternal and fetal thyroid function.
Ultrasonographic findings in Autoimmune Thyroid Disease
Figure 1:
Diffuse hypoechogenicity and heterogeneity associated with autoimmune thyroid disease.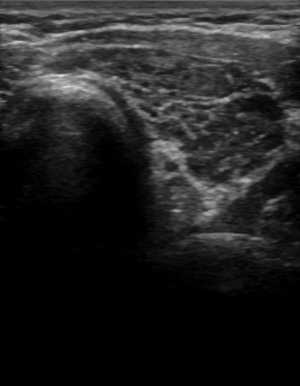
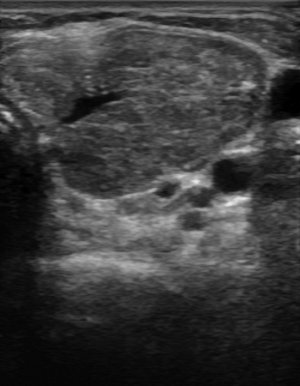
Another early clue to the presence of a diffuse thyroid disorder is a thickened isthmus. The normal isthmus size in the transverse view is less than 3-4 mm; a measurement of >5mm may be an indication of an underlying disorder[8]. While not specific for AITD, it can be an early harbinger.
As a result of the lymphocytic involvement, patients with autoimmune thyroid disease may also have reactive lymphoid hyperplasia[9]. While this reactive lymphadenopathy is more commonly associated with patients with Hashimoto’s thyroiditis, it may also be seen in Graves’ disease. More easily visualized lateral to the carotid arteries, reactive nodes may also be found inferior and superior to the thyroid gland in the ‘central neck’[8]. The distribution of these nodes symmetrically in the neck and their reactive appearance are reassuring features. The nodes may be enlarged up to 1-2 cm but have a characteristic fusiform shape (relatively flat with width greater than two times the height) and retain their normal architecture with a prominent hilar stripe[9]. Absence of these reassuring features or any doubts about the benignity of the nodes should prompt the clinician to consider FNA to determine their malignant potential[10].
Graves’ disease versus Hashimoto’s thyroiditis
Figure 2:
Color-flow Doppler analysis of Graves’ disease.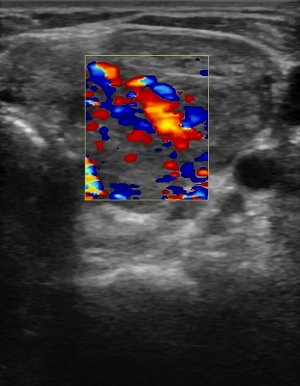
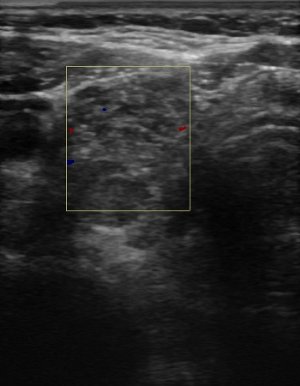
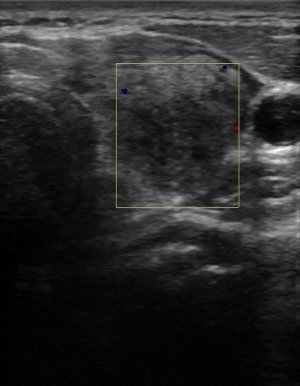
Because the amount of vascularity in a gland may be a qualitative parameter, peak systolic velocity (PSV) is a convenient quantitative index that is simple to measure with modern US machines[11]. Medium-sized arteries within the thyroid gland or in the inferior thyroidal artery are examined to determine the velocity of flow. Absolute values vary for each study, but the comparison to normal healthy controls reveals a marked difference in rates. For example, one group found that mean normal intraparenchymal peak systolic velocity was 4.8 ± 1.2cm/s compared to patients with untreated GD who had velocities of 15 ± 3cm/s[14].
Ultrasonographic findings in toxic adenomas versus cold nodules
Vascular flow has also been used to distinguish toxic adenomas from cold nodules[11],[15]. The pattern associated with autonomous adenomas is controversial, however. Some studies find that perinodular flow is increased in toxic nodules compared to cold nodules[14],[16],[17], whereas others have found that intranodular flow is increased in functioning adenomas[15],[18]. Still others[19],[20] have found that both intra- and perinodular flow is increased in toxic nodules. It is important to note, though, that malignant thyroid nodules may also have increased Doppler flow within them[21]. Vascular analysis may be a helpful component of the overall evaluation of thyroid nodules, but its use as a single determinant in assessing the risk of malignancy or autonomy of a nodule remains limited.
Ultrasound findings with destructive thyroiditis
Figure 3:
Ultrasound findings with subacute thyroiditis. Acute phase of subacute thyroiditis.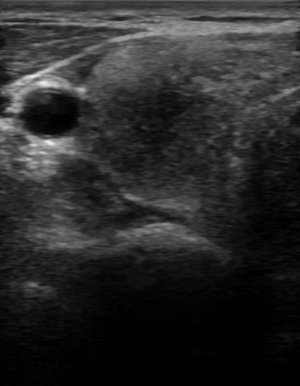
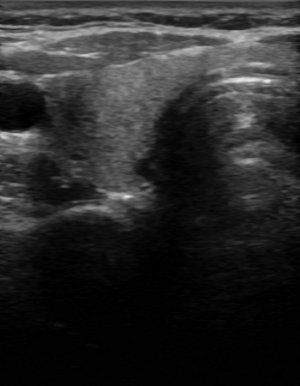
Amiodarone
Amiodarone-induced thyrotoxicosis (AIT) may be divided into two main forms: type 1, a form of iodine-induced hyperthyroidism (Jod-Basedow phenomenon), which is treated with antithyroid drugs; and type 2, a drug-induced destructive thyroiditis, which is treated with glucocorticoids[29]. Because the treatment varies with the type of thyroiditis, distinction of these conditions is important. Radioiodine uptake, in theory, should help discriminate between these two entities. In reality, however, the values of iodine uptake can overlap between type 1 and type 2 AIT[30],[31]. Ultrasound, specifically color-flow Doppler, has been studied to differentiate these two disorders[30],[32],[33],[34]. Patients with type 1 AIT are found to have increased blood flow through the inferior thyroidal artery[34], a vascular pattern similar to untreated Graves’ disease[32], or a hypervascular nodular pattern[33]. Conversely, patients with type 2 AIT had absent or markedly decreased vascularity on color-flow Doppler[30],[32],[33],[34]. One retrospective study found that CFDS was able to distinguish type 1 and 2 in 80% of cases[35]. Ultrasound, therefore, may be considered in the initial workup of patients suspected of having AIT.
Nuclear imaging for hyperthyroidism
Though it was long recognized that iodine is avidly taken up in large goiters, the clinical utility of iodine for diagnostic and therapeutic measures was not realized until isotopes were formulated and the emission of beta and gamma rays in situ was captured. Several isotopic variations were created in the late 1930s and testing in rabbits revealed its efficacy for the treatment of hyperthyroidism[36]. In the early 1940s radioiodine was being used in humans for both the diagnosis and treatment of Graves’ disease[37]. The overwhelming success of this treatment is based on the fact that normal glands take up relatively small amounts of the isotope, converging greater concentrations of radiation in the hyperplastic portions of the gland.The use of radionuclide imaging in the diagnostic workup of hyperthyroidism remains widely popular amongst Endocrinologists and primary care physicians, particularly in the United States. In fact, radionuclide scanning is the recommended initial step in the diagnosis of patients with hyperthyroidism in some guidelines[38], even though the diagnosis can often be made on physical examination alone. There is no doubt that it is a highly effective method for diagnosing Graves’ disease, one study found that 123I scanning had a specificity of 96% when >20% uptake was seen at 5-6 hours[39].
Comparison of ultrasound and nuclear imaging
While there is clearly an established role for nuclear scintigraphy in the diagnosis of hyperthyroidism, there are also important limitations to its use (see Table 1). When compared with ultrasound, scintigraphy is significantly less sensitive for diagnosing thyroid nodules[40],[41]. In one prospective study of 426 patients with Graves’, ultrasound identified 68 (16%) thyroid nodules, whereas pertechnetate scanning only detected 9 (2.1%) nodules (p<0.001)[40]. In another study, US uncovered nodules that palpation and scintigraphy failed to identify in 12.1% of patients[41]. It is important to consider the size of the nodules as radionuclide scanning may not distinguish thyroid nodules measuring less than 1-1.5 cm[31]. However, even when considering only those nodules measuring over a centimeter, scintigraphy performs poorly, it was only able to localize 30% of cold nodules[40]. In this same cohort, 30/68 (47.7%) patients were diagnosed with thyroid cancer, only four of which were found by scintigraphy[40].Imaging modality | Advantages | Disadvantages | Accuracy[27],[40] |
---|---|---|---|
Thyroid ultrasound |
|
|
|
Thyroid nuclear scan |
|
|
|
Another drawback of radionuclide scanning is the significant cost. For an ultrasound in the United States, physicians bill the patients or their insurance companies $401; actual Medicare reimbursement is $115 (personal communication with medical billing department at Ohio State University). A 123I uptake and scan will incur a cost of $1,049; Medicare reimburses $219.49. Pertechnetate scanning is slightly less expensive at $928; the reimbursement rate from Medicare is $132.79. One study in Italy performed a cost analysis comparing pertechnetate scanning and US in the initial workup of Graves’ disease and found that the total cost to obtain a diagnosis by US for all patients in the study was €14,645.34 compared to €19,922.71 for scintigraphy[40]. The authors recommend US as a first step in the diagnosis of all hyperthyroid patients, and that scintigraphy should be limited only to uncommon cases where the diagnosis cannot be made with clinical clues and laboratory data[40].
Because it has traditionally played such a large role in the diagnosis of hyperthyroidism, nuclear scanning is readily available in most areas. Routine use of ultrasound for the evaluation of thyrotoxicosis, on the other hand, is significantly limited by a lack of familiarity with its applicability in this condition. More education is needed to acquaint providers with the role of US in the diagnosis and management of thyrotoxicosis.
Increasingly it is being recognized that US has a significant role in the diagnosis of Graves’ disease; it is a convenient, inexpensive, and noninvasive technique to determine the underlying pathophysiology of thyrotoxicosis[42]. Furthermore, US and scintigraphy are equally accurate at diagnosing Graves’ disease; in one large study, each were able to make the correct diagnosis in 95.2% and 97.4% of patients, respectively (p=0.763)[40]. Ultrasonography offers the additional advantage of making the diagnosis without exposing the patient to ionizing radiation. One recent study found that thyroid scintigraphy and uptake studies did not influence the diagnosis or treatment outcomes in most cases of hyperthyroidism[43]. The authors recommend against its routine use, except when clinical features, laboratory assays, and ultrasound exams are not diagnostic, to reduce unnecessary costs and exposure to radioisotopes[43].
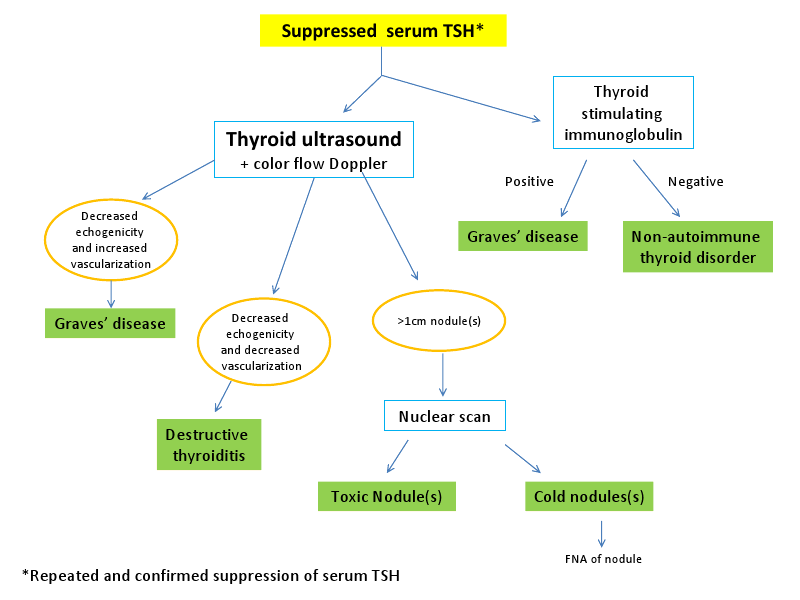
Utility of ultrasonography for post-treatment follow up in Graves’ disease
Up to 60% of patients will achieve a remission of their Graves’ disease with medical therapy alone, but the course of the disease is heterogeneous and difficult to predict[44],[45]. A clinical tool that could distinguish which patients will go into remission would be extremely useful during the early phases of the disease to help the patient and clinician decide which therapy to choose. Patients who are highly likely to go into remission may elect medical management initially so that lifelong therapy with thyroid hormone supplementation could be avoided, as would be expected after radioiodine treatment or surgery. Thyroid appearance on ultrasound has been used as a tool to predict which patients with Graves’ disease will have a remission. Investigators have found that those patients requiring active antithyroid drug treatment had significantly lower thyroid echogenicity compared with those whose disease was inactive (p<0.001)[46],[47]. Two prospective trials have confirmed that echogenicity after thionamide treatment is predictive of remission[48],[49]. One group found that a normal thyroid echogenicity score at withdrawal of methimazole had a higher specificity (95%) than TRAb values (86%) for prediction of remission two years after initiation of therapy[49]. Likewise, another study revealed a relationship between thyroid echogenicity and the clinical course and immunologic parameters in Graves’ disease[48]. Patients who had a hypoechoic, micronodular appearance on US were more likely to have a high TRAb titer and were at a higher risk of recurrence of hyperthyroidism after treatment withdrawal[48].Vascularity has also been investigated to establish its value in predicting the relapse of hyperthyroidism in Graves’ patients after withdrawal of antithyroid drugs[50],[51],[52]. One group found that patients who were able to be withdrawn from thionamides had significantly lower thyroid blood flow and vessel numbers compared to the untreated and the active treatment groups[51]. Furthermore, these vascular indices were significantly higher in those who relapsed, compared to those in a stable remission[51]. This relationship retained its predictive value on multivariate analysis. Similarly, another group found significantly increased blood flow parameters (including volume flow, systolic maximum velocity, end-diastolic velocity, and resistive index) in patients with active hyperthyroidism before treatment and in euthyroid patients who had a relapse after withdrawal of antithyroid treatment versus normal controls[53]. On the other hand, those patients who remained in stable remission had no significant difference in the blood flow parameters compared to normal controls[53]. Looking only at patients about to be withdrawn from thionamide therapy, one group found that higher blood flow at the inferior thyroid artery was an early predictor for Graves’ disease relapse[52]. Based on these studies, it appears that thyroid blood flow changes may be related to the course of Graves’ disease.
The use of ultrasonography in pregnancy
Nuclear imaging is contraindicated during pregnancy because of its toxicity to the developing fetal thyroid. Ultrasound therefore is a useful tool to distinguish between various forms of hyperthyroidism during pregnancy. One group showed that thyroid volume is significantly higher in patients with Graves’ disease compared with gestational thyrotoxicosis or destructive thyroiditis (18.9 ± 1.5cm3 vs. 12.1 ± 2.4cm3, p<0.05)[54]. Thyroid vascularity and inferior thyroidal artery flow velocity were also greater in the Graves’ disease group compared with the non-Graves’ patients (p<0.05)[54].Although Graves’ disease during pregnancy is rare, affecting only 0.2% of pregnancies, recognition of the effects of maternal TSH receptor antibodies on the developing fetus is critical[55]. Maternal TSH receptor antibodies freely cross the placenta and can stimulate the fetal thyroid gland in the later stages of the pregnancy, causing hyperthyroidism[56]. Conversely, antithyroid drugs also cross the placenta and can induce fetal hypothyroidism[55]. Ultrasound is a noninvasive test that can detect fetal thyroid disorders; in conjunction with cord blood tests, one study found that detection of a fetal goiter on US has a sensitivity of 92% and a specificity of 100% for identifying clinically relevant fetal thyroid dysfunction[55]. A second study utilized serial in utero US alone to measure fetal size in mothers with Graves’ disease to see if they could avoid the use of invasive blood sampling[57]. When thyroid size was above the 95th percentile for gestational age, maternal dose of thionamide was reduced. The three fetuses whose thyroid volume declined to the normal range were born euthyroid. In the two fetuses whose thyroid size was unaffected by reduction in dosage, both were thyrotoxic at birth. The authors suggest that US is an effective method to monitor maternal antithyroid drug dose, preventing intrauterine hypothyroidism. If the goiter is not reduced in size by dose adjustment of the thionamide, they suggest that transplacental passage of thyroid-stimulating antibodies may be causing thyrotoxicosis in the fetus. Further, they recommend consideration of fetal thyroid status by cord blood sampling to determine if the fetus is thyrotoxic[57]. Treatment consists of administering methimazole to the mother and reassessing fetal tachycardia and goiter size[58]. A recent study confirmed that fetal thyroid goiter can be detected by US and that a reduction in dose of PTU prevented development of neonatal hypothyroidism[59]. It is now recommended that fetal US should be performed to look for evidence of fetal thyroid dysfunction in women with an elevated TRAb or in those treated with antithyroid drugs[60].
Summary
Ultrasound has myriad uses in the evaluation of patients with hyperthyroidism. It is a sensitive and specific test for diagnosing various causes of hyperthyroidism, particularly during pregnancy. In experienced hands, US can help predict relapse or remission of Graves’ disease after medical therapy, aiding the clinician in determining the best form of therapy. Finally, it can help predict fetal thyroid dysfunction without increasing the risk of cordocentesis-related complications. We therefore recommend its use in the initial workup of patients with thyrotoxicosis.- Shokurov NN. [Ultrasound in diagnosis of certain diseases of the thyroid]. Eksp Khir Anesteziol. 1966;11(2):31-4.
- Singer PA, Cooper DS, Daniels GH, et al. Treatment guidelines for patients with thyroid nodules and well-differentiated thyroid cancer. American Thyroid Association. Arch Intern Med. 1996;156(19):2165-72.
- Brander A, Viikinkoski P, Tuuhea J, et al. Clinical versus ultrasound examination of the thyroid gland in common clinical practice. J Clin Ultrasound. 1992;20(1):37-42.
- Pedersen OM, Aardal NP, Larssen TB, et al. The value of ultrasonography in predicting autoimmune thyroid disease. Thyroid. 2000;10(3):251-9.
- Raber W, Gessl A, Nowotny P, Vierhapper H. Thyroid ultrasound versus antithyroid peroxidase antibody determination: a cohort study of four hundred fifty-one subjects. Thyroid. 2002;12(8):725-31.
- Yeh HC, Futterweit W, Gilbert P. Micronodulation: ultrasonographic sign of Hashimoto thyroiditis. J Ultrasound Med. 1996;15(12):813-9.
- Rago T, Chiovato L, Grasso L, et al. Thyroid ultrasonography as a tool for detecting thyroid autoimmune diseases and predicting thyroid dsfunction in apparently healthy subjects. J Endocrinol Invest. 2001;24(10):763-9.
- Kharchenko VP. Ultrasound diagnostics of thyroid diseases. New York: Springer; 2010.
- Paksoy N, Yazal K. Cervical lymphadenopathy associated with Hashimoto's thyroiditis: an analysis of 22 cases by fine needle aspiration cytology. Acta Cytol. 2009;53(5):491-6.
- Sipos JA. Advances in ultrasound for the diagnosis and management of thyroid cancer. Thyroid. 2009;19(12):1363-72.
- Erdogan MF, Anil C, Cesur M, et al. Color flow Doppler sonography for the etiologic diagnosis of hyperthyroidism. Thyroid. 2007;17(3):223-8.
- Ralls PW, Mayekawa DS, Lee KP, et al. Color-flow Doppler sonography in Graves disease: "thyroid inferno". AJR Am J Roentgenol. 1988;150(4):781-4.
- Vitti P, Rago T, Mazzeo S, et al. Thyroid blood flow evaluation by color-flow Doppler sonography distinguishes Graves' disease from Hashimoto's thyroiditis. J Endocrinol Invest. 1995;18(11):857-61.
- Bogazzi F, Bartalena L, Brogioni S, et al. Thyroid vascularity and blood flow are not dependent on serum thyroid hormone levels: studies in vivo by color flow doppler sonography. Eur J Endocrinol. 1999;140(5):452-6.
- Becker D, Bair HJ, Becker W, et al. Thyroid autonomy with color-coded image-directed Doppler sonography: internal hypervascularization for the recognition of autonomous adenomas. J Clin Ultrasound. 1997;25(2):63-9.
- Fobbe F, Finke R, Reichenstein E, et al. Appearance of thyroid diseases using colour-coded duplex sonography. Eur J Radiol. 1989;9(1):29-31.
- Lupi A, Cerisara D, Orsolon P, et al. Thyroid nodules and Doppler ultrasonography. A new element for an old puzzle? Minerva Endocrinol. 1999;24(1):7-10.
- Clark KJ, Cronan JJ, Scola FH. Color Doppler sonography: anatomic and physiologic assessment of the thyroid. J Clin Ultrasound. 1995;23(4):215-23.
- Gimondo P, Pizzi C, Gimondo S, Messina G. [Ultrasonography, with Doppler color, and cytologic correlations in Plummer's disease]. Radiol Med. 1998;95(3):193-8.
- Boi F, Loy M, Piga M, et al. The usefulness of conventional and echo colour Doppler sonography in the differential diagnosis of toxic multinodular goitres. Eur J Endocrinol. 2000;143(3):339-46.
- Shimamoto K, Endo T, Ishigaki T, et al. Thyroid nodules: evaluation with color Doppler ultrasonography. J Ultrasound Med. 1993;12(11):673-8.
- Shigemasa C, Teshima S, Taniguchi S, et al. Pertechnetate thyroid uptake is not always suppressed in patients with subacute thyroiditis. Clin Nucl Med. 1997;22(2):109-14.
- Bennedbaek FN, Hegedus L. The value of ultrasonography in the diagnosis and follow-up of subacute thyroiditis. Thyroid. 1997;7(1):45-50.
- Park SY, Kim EK, Kim MJ, et al. Ultrasonographic characteristics of subacute granulomatous thyroiditis. Korean J Radiol. 2006;7(4):229-34.
- Brander A. Ultrasound appearances in de Quervain's subacute thyroiditis with long-term follow-up. J Intern Med. 1992;232(4):321-5.
- Hari Kumar KV, Pasupuleti V, Jayaraman M, et al. Role of thyroid Doppler in differential diagnosis of thyrotoxicosis. Endocr Pract. 2009;15(1):6-9.
- Kurita S, Sakurai M, Kita Y, et al. Measurement of thyroid blood flow area is useful for diagnosing the cause of thyrotoxicosis. Thyroid. 2005;15(11):1249-52.
- Uchida T, Takeno K, Goto M, et al. Superior thyroid artery mean peak systolic velocity for the diagnosis of thyrotoxicosis in Japanese patients. Endocr J. 2010;57(5):439-43.
- Bogazzi F, Bartalena L, Martino E. Approach to the patient with amiodarone-induced thyrotoxicosis. J Clin Endocrinol Metab. 2010;95(6):2529-35.
- Bogazzi F, Martino E, Dell'Unto E, et al. Thyroid color flow doppler sonography and radioiodine uptake in 55 consecutive patients with amiodarone-induced thyrotoxicosis. J Endocrinol Invest. 2003;26(7):635-40.
- Sarkar SD. Benign thyroid disease: what is the role of nuclear medicine? Semin Nucl Med. 2006;36(3):185-93.
- Bogazzi F, Bartalena L, Brogioni S, et al. Color flow Doppler sonography rapidly differentiates type I and type II amiodarone-induced thyrotoxicosis. Thyroid. 1997;7(4):541-5.
- Loy M, Perra E, Melis A, et al. Color-flow Doppler sonography in the differential diagnosis and management of amiodarone-induced thyrotoxicosis. Acta Radiol. 2007;48(6):628-34.
- Macedo TA, Chammas MC, Jorge PT, et al. Differentiation between the two types of amiodarone-associated thyrotoxicosis using duplex and amplitude Doppler sonography. Acta Radiol. 2007;48(4):412-21.
- Eaton SE, Euinton HA, Newman CM, et al. Clinical experience of amiodarone-induced thyrotoxicosis over a 3-year period: role of colour-flow Doppler sonography. Clin Endocrinol (Oxf). 2002;56(1):33-8.
- Sawin CT, Becker DV. Radioiodine and the treatment of hyperthyroidism: the early history. Thyroid. 1997;7(2):163-76.
- Hertz S, Roberts A. Radioactive Iodine as an Indicator in Thyroid Physiology. V. The Use of Radioactive Iodine in the Differential Diagnosis of Two Types of Graves' Disease. J Clin Invest. 1942;21(1):31-2.
- Baskin HJ, Cobin RH, Duick DS, et al. American Association of Clinical Endocrinologists medical guidelines for clinical practice for the evaluation and treatment of hyperthyroidism and hypothyroidism. Endocr Pract. 2002;8(6):457-69.
- Hennessey JV, Berg LA, Ibrahim MA, Markert RJ. Evaluation of early (5 to 6 hours) iodine 123 uptake for diagnosis and treatment planning in Graves' disease. Arch Intern Med. 1995;155(6):621-4.
- Cappelli C, Pirola I, De Martino E, et al. The role of imaging in Graves' disease: a cost-effectiveness analysis. Eur J Radiol. 2008;65(1):99-103.
- Torizuka T, Kasagi K, Hatabu H, et al. Clinical diagnostic potentials of thyroid ultrasonography and scintigraphy: an evaluation. Endocr J. 1993;40(3):329-36.
- Vitti P. Grey scale thyroid ultrasonography in the evaluation of patients with Graves' disease. Eur J Endocrinol. 2000;142(1):22-4.
- Okosieme OE, Chan D, Price SA, et al. The utility of radioiodine uptake and thyroid scintigraphy in the diagnosis and management of hyperthyroidism. Clin Endocrinol (Oxf). 2010;72(1):122-7.
- Ishtiaq O, Waseem S, Haque MN, et al. Remission of Grave's disease after oral anti-thyroid drug treatment. J Coll Physicians Surg Pak. 2009;19(11):690-3.
- Cappelli C, Gandossi E, Castellano M, et al. Prognostic value of thyrotropin receptor antibodies (TRAb) in Graves' disease: a 120 months prospective study. Endocr J. 2007;54(5):713-20.
- Schiemann U, Gellner R, Riemann B, et al. Standardized grey scale ultrasonography in Graves' disease: correlation to autoimmune activity. Eur J Endocrinol. 1999;141(4):332-6.
- Vitti P, Rago T, Mancusi F, et al. Thyroid hypoechogenic pattern at ultrasonography as a tool for predicting recurrence of hyperthyroidism after medical treatment in patients with Graves' disease. Acta Endocrinol (Copenh). 1992;126(2):128-31.
- Baldini M, Orsatti A, Bonfanti MT, et al. Relationship between the sonographic appearance of the thyroid and the clinical course and autoimmune activity of Graves' disease. J Clin Ultrasound. 2005;33(8):381-5.
- Zingrillo M, D'Aloiso L, Ghiggi MR, et al. Thyroid hypoechogenicity after methimazole withdrawal in Graves' disease: a useful index for predicting recurrence? Clin Endocrinol (Oxf). 1996;45(2):201-6.
- Castagnone D, Rivolta R, Rescalli S, et al. Color Doppler sonography in Graves' disease: value in assessing activity of disease and predicting outcome. AJR Am J Roentgenol. 1996;166(1):203-7.
- Baldini M, Castagnone D, Rivolta R, et al. Thyroid vascularization by color doppler ultrasonography in Graves' disease. Changes related to different phases and to the long-term outcome of the disease. Thyroid. 1997;7(6):823-8.
- Ueda M, Inaba M, Kumeda Y, et al. The significance of thyroid blood flow at the inferior thyroid artery as a predictor for early Graves' disease relapse. Clin Endocrinol (Oxf). 2005;63(6):657-62.
- Varsamidis K, Varsamidou E, Mavropoulos G. Doppler ultrasonography in predicting relapse of hyperthyroidism in Graves' disease. Acta Radiol. 2000;41(1):45-8.
- Hari Kumar KV, Vamsikrishna P, Verma A, et al. Evaluation of thyrotoxicosis during pregnancy with color flow Doppler sonography. Int J Gynaecol Obstet. 2008;102(2):152-5.
- Luton D, Le Gac I, Vuillard E, et al. Management of Graves' disease during pregnancy: the key role of fetal thyroid gland monitoring. J Clin Endocrinol Metab. 2005;90(11):6093-8.
- Zakarija M, McKenzie JM. Pregnancy-associated changes in the thyroid-stimulating antibody of Graves' disease and the relationship to neonatal hyperthyroidism. J Clin Endocrinol Metab. 1983;57(5):1036-40.
- Cohen O, Pinhas-Hamiel O, Sivan E, et al. Serial in utero ultrasonographic measurements of the fetal thyroid: a new complementary tool in the management of maternal hyperthyroidism in pregnancy. Prenat Diagn. 2003;23(9):740-2.
- Patil-Sisodia K, Mestman JH. Graves hyperthyroidism and pregnancy: a clinical update. Endocr Pract. 2010;16(1):118-29.
- Rosenfeld H, Ornoy A, Shechtman S, Diav-Citrin O. Pregnancy outcome, thyroid dysfunction and fetal goitre after in utero exposure to propylthiouracil: a controlled cohort study. Br J Clin Pharmacol. 2009;68(4):609-17.
- Abalovich M, Amino N, Barbour LA, et al. Management of thyroid dysfunction during pregnancy and postpartum: an Endocrine Society Clinical Practice Guideline. J Clin Endocrinol Metab. 2007;92(8 Suppl):S1-47.